The Unbearable Lightness of Being Microscopic
INTRODUCTION
Microorganisms are often viewed and taught as extremely simple creatures lacking much interest except for pathological or environmental significance. David B. Dusenbery, professor emeritus of biology at Georgia Tech, has undertaken the challenging task of examining physical principles governing microorganisms. The book is divided into five sections: background, physical consequences, consequences for locomotion, consequences for orientation to stimulus gradients, and consequences for interactions between organisms. The Background section consists of two chapters on microscopy and fluids, respectively. These two chapters are crucial not only to provide readers physical background but also to get them used to the author's writing style—one that oscillates between biological background information and physical principles supported by equations and mathematical data.
LOCOMOTION AND INTERACTIONS
Section III provides the basics of how microorganisms transport chemicals and obtain information by detecting signals. Chapter 9 argues that effective mechanisms for chemical transport are essentially different for microbes and provides effective and elegant mathematical characterization of diffusion. Although so much mathematical information could prove challenging for many biologists, the author does a great job of simplifying complex mathematical formulas and principles. Sensory mechanisms are discussed in great detail along with their significance for movement in a favorable direction. Dusenbery goes into much detail to support the notion that although Brownian motion can result in erratic movement, it is sometimes necessary for effective dispersal of their species. I learned from reading this book that a spherical shape takes the least amount of time to diffuse over a given distance, which may explain why most viruses have spherical or sphere-like shapes.
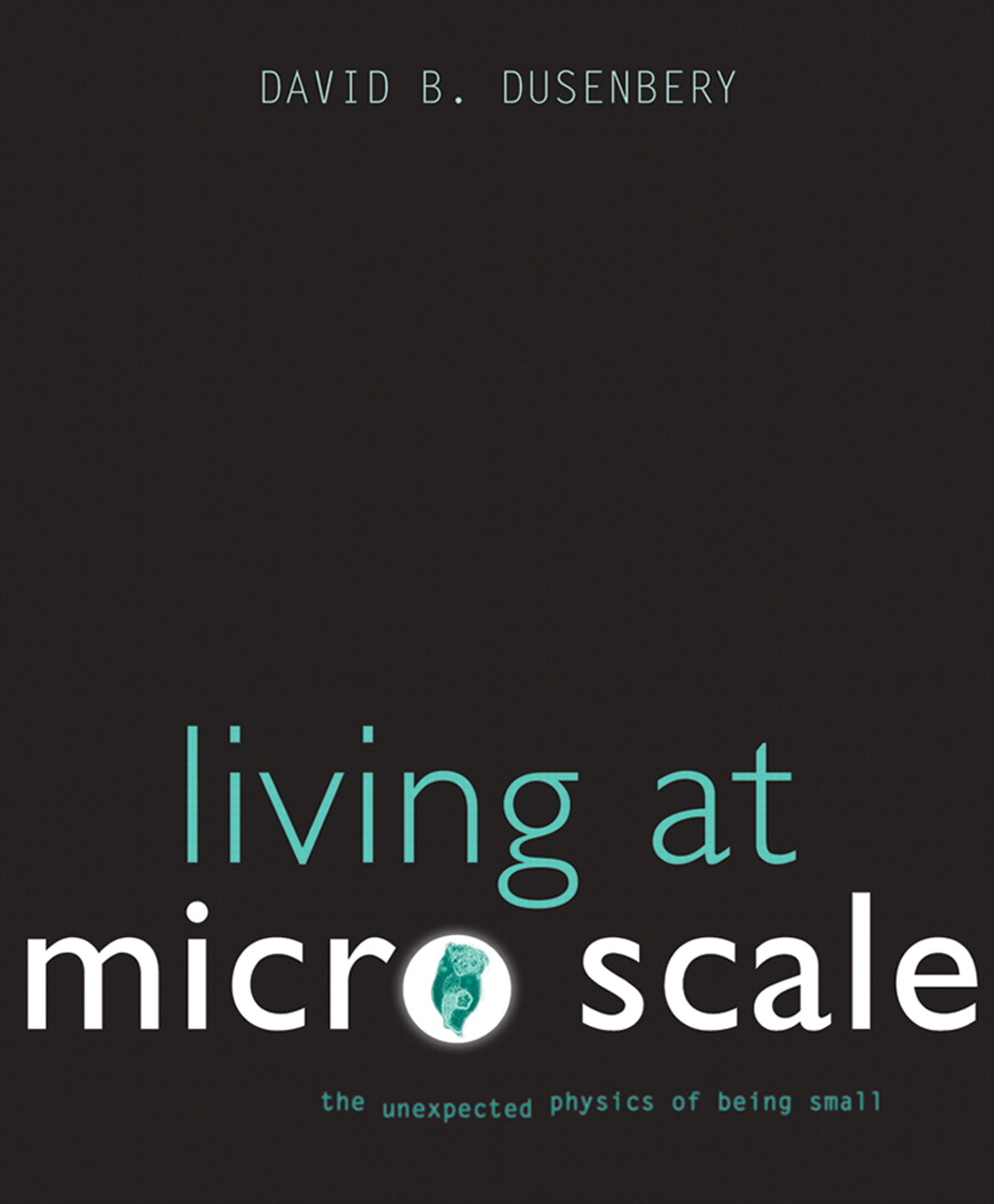
The sedimentation rate can affect nutrient uptake, but for microorganisms, swimming still provides a faster method for locomotion. Notable exceptions are diatoms, which cannot swim due to their dense shell, and benefit from access to nutrients by sedimentation. However, using sedimentation to move through a fluid provides a disadvantage because the movement is toward the center, which leads to less access to oxygen. On the other hand, anaerobic microorganisms benefit from having a high sedimentation rate. The author constantly brings some evolutionary adaptations to his narrative, which in turn may explain some of the mathematical differences presented for a given mechanism.
Swimming gets a lot of coverage in the book. It proves to be a more efficient mechanism for propulsion in microorganisms, and these use cylindrical appendages due to a low Reynolds number (an essential parameter in heat transfer and fluid mechanics). Thus, cylindrical appendages such as cilia and flagella are ideal for swimming. In eukaryotes, the cilia and flagella also allow them to change the direction of orientation. A single flagellum generates sufficient thrust, but its bending causes the microbe's body to wobble, which may explain why some larger microorganisms use cilia rather than flagella for their motion. The size limit for locomotion covered in Chapter 15 proposes that spatial comparison mechanisms may have evolved into temporal mechanisms. I found this particular section confusing and lacking in more detailed explanations.
Locomotion is essential for microorganisms to respond to a stimulus gradient. Shape has a determining effect on the bacterium's ability to detect a stimulus gradient; thus, a rod shape helps bacteria with nutrient acquisition since it is optimal in the direction of swimming. This argument may explain why so many bacteria are rod-shaped, which in turn may be based on mechanisms of cell division and growth.
The last section, called “Encounter Rates,” becomes repetitive as the author goes into concepts previously discussed while adding very little new information. The chapter on predation did not meet my expectations, as there was no mention of phagocytosis or similar engulfment phenomena. Similarly, a chapter on pheromone attraction did not seem to fit with the rest of the book's narrative. There is one last chapter on gametes, which suggests that large organisms rely on sperm and eggs possibly to have more effective gamete encounters while reproducing at lower population densities.
CONCLUSIONS
Living at Micro Scale represents a milestone by intersecting microbiology, evolutionary biology, and physics. The author pays much attention to details pertaining to mathematical principles, which may prevent prospective readers from becoming engaged. However, any instructor wishing to bring an interdisciplinary approach to a cell biology, microbiology, or physiology course will find plenty of material and resources to bring physical parameters of various microscale phenomena. Although the narrative may be repetitive at times, Dusenbery should be commended for bringing us to confront the notion that microorganisms follow complex physical principles, which in turn may have some significant evolutionary significance. As he states, the physics of microorganisms is different from the physics we are used to, with no role for inertia and a more prominent role for Brownian motion. After reading this book, I gained a greater understanding of why microorganisms are the way they are—which indicates that it is not easy being small.