Interdisciplinarity: The Right People, a Supportive Place, and a Program Emerges
Twenty-first-century biology is inherently interdisciplinary. Every aspect of biology, from molecules to organisms to ecosystems, is richly informed by the physical, mathematical, and computational sciences. Interdisciplinarity is a buzzword in biology education, and understandably so, for the major scientific challenges of the 21st century—climate change, global health, personalized medicine, and genetic engineering, to name a few—require insights from across the natural and mathematical sciences.
It is both an exciting and daunting time for biology educators—exciting because of the vast opportunity for important new discoveries that will come about in an interdisciplinary context, yet daunting for many current biology educators who were not trained within such a framework.
The call for transformation of the undergraduate biology experience to better address interdisciplinarity is not new. It has been 10 years since BIO2010: Transforming Undergraduate Education for Future Research Biologists (National Research Council, 2003) channeled the growing awareness of how technological advances blurred traditional disciplinary boundaries, challenging science educators to modify how and what we teach. Recently, the American Association for the Advancement of Science (2011) reissued the call in Vision and Change in Undergraduate Biology Education: A Call to Action. If our experience is any indication, even with broad interdisciplinary buy in, understanding how best to implement interdisciplinarity into the undergraduate science and mathematics experience is not always easy.
At St. Olaf College, a liberal arts college with an undergraduate-only population of ∼3000, we are fortunate in that our relatively small size allows for a certain degree of nimbleness as we consider changes that foster interdisciplinarity. We are also fortunate to have a clear sense of opportunity: students come to us predominantly from disciplinary-based high school science/mathematics education programs (see important companion essay by Barbara Nagle [2013] about how this is changing), amplifying our potential for transformation to a more interdisciplinary way of understanding the natural and mathematical sciences. In this essay, we share some perspectives and examples that have emerged for us over the past 10 years or so as we have attempted to offer a broadly interdisciplinary environment in which our students and faculty explore and understand biology.
THE THREE “P”S: PEOPLE, PLACE, AND PROGRAM
Institutions of higher education might be thought of as consisting of the three “P”s—people, place, and program. Certain people (faculty, staff, administrators, regents), working within the opportunities and constraints afforded by a place (buildings and grounds), do their best to provide meaningful educational programs (curricular, cocurricular, extracurricular) for students. For us at St. Olaf College, the key to success in transforming to a more interdisciplinary program resided in a focus on the people and the place.
GET THE RIGHT PEOPLE ON THE BUS
Jim Collins (2001), in his book Good to Great, describes how companies that made a successful shift from being “good” to being “great” focused on getting the right people “on the bus,” on focusing on the “who” before the “what.” To a certain extent, this was true in our desire to become more interdisciplinary. From around 2001 onward, every tenure-track search conducted in the natural and mathematical sciences was conducted with an eye toward interdisciplinarity. Job descriptions were explicit about our interest in interdisciplinary approaches. Search committees contained voting members from other departments. Interdisciplinarity was routinely discussed during interviews. Every on-campus interview included a division-wide reception at which the candidate met faculty outside the department(s) into which she or he would be hired.
Since 2001, St. Olaf has brought in new faculty members who carry descriptors such as biophysical chemist, biogeochemist, behavioral neuroscientist, mathematical biologist, bioanalytical chemist, molecular ecologist, cognitive neuroscientist, neurobiologist, bioinformaticist, and biostatistician. Even when we hired into positions that were advertised in more traditional areas, such as experimental physics or pure mathematics, faculty members who were keen about interdisciplinarity were hired. In many cases, these faculty members were quick to develop thoughtful, cross-departmental research collaborations, even though they were not hired specifically for their interdisciplinary expertise. When the needs and timing were aligned, we made joint appointments, one in chemistry/biology, one in biology/environmental studies, and a just-completed one in biology/physics/neuroscience.
PLACE MATTERS
Winston Churchill said, “We shape our buildings, and afterward our buildings shape us.” At St. Olaf College, we were fortunate to be in the design phase for new science and mathematics facilities at the same time we were addressing the programmatic need for greater interdisciplinarity. After much debate, we decided to organize our new Regents Hall of Natural and Mathematical Sciences (opened Fall 2008; see www.stolaf.edu/regentshall) in a manner that fosters interdisciplinarity, while sustaining disciplinary foundations. There are deliberately designed interdisciplinary spaces throughout the building, including clusters dedicated to neuroscience, biomolecular science, and the environmental sciences, but one can also find disciplinary “homes” in the building. Faculty offices and shared research laboratories are organized by common interests, regardless of their disciplinary home, an arrangement that makes interactions among faculty from different departments much more likely than our old Science Center, where each department occupied its own floor, and the natural sciences and mathematics departments were scattered among three different buildings. A detailed description of the advantages of this arrangement on our neuroscience program is available elsewhere (Muir and Van Wylen, 2009).
There is little doubt that Regents Hall has been an enormous boost to our interdisciplinary initiatives. However, as suggested by Churchill, the boost was synergistic: having a clear programmatic vision for interdisciplinarity informed the design of Regents Hall, and living in an integrated building now naturally shapes and supports our interdisciplinary initiatives (e.g., faculty hires, undergraduate research, curriculum).
Does an interdisciplinary layout influence the perceptions of students about the practice of science? Can spaces designed with the intent to promote visual connectedness between disciplines help students make those connections for themselves? We assessed these questions by comparing student surveys administered in 2008, when we still resided in our old Science Center, and again in 2009, after we had moved into Regents Hall (Van Wylen and Walczak, 2011). Students enrolled in biology, chemistry, physics, and psychology lab courses were asked to respond to a series of statements on a 5-point Likert scale with strongly agree, agree, neutral, disagree, and strongly disagree options. The responses to two statements (see Figure 1) are of particular interest here (graphed as percentage of respondents who strongly agree [SA] or agree [A] with the statement).
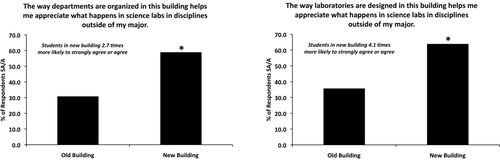
Figure 1. Percent of survey respondents who strongly agree or agree (SA/A) with the indicated statements. See text for details.
These data suggest that students who practice science in our new Regents Hall have a greater appreciation of what happens in science labs in disciplines outside their majors as compared with students who worked in our old science building. This apparently is a result of both the mixing of departments throughout the building (Figure 1, left) and by the way the laboratories are designed with ample opportunity to observe science across the disciplines (Figure 1, right).
THE EMERGING PROGRAM
Over time, with the right people “on the bus,” several interdisciplinary curricular initiatives emerged. Some examples are discussed in the following sections.
Integrated Introductory Chemistry/Biology Course
With funding from a grant from the Howard Hughes Medical Institute (HHMI), our biology and chemistry departments developed an integrated three-course chemistry and biology sequence (known as Ch/Bi) for first-year students. The integrated Ch/Bi sequence covers the content of our first year of introductory chemistry and our first semester of introductory biology (cell biology and genetics). HHMI funding provided released time to a group of biologists and chemists to research the current state of biology–chemistry integration on college campuses; visit courses in the “other” discipline; and then develop, teach, and assess pilot sections of the course. The goals of Ch/Bi were to help students:
Avoid disciplinary compartmentalization of their knowledge, which often makes that knowledge inaccessible during a course in another discipline (Bransford and Cocking, 2000). Perhaps readers have identified the same issue: consider a student who started in chemistry and is now in biology but who seems to have no knowledge of the Beer-Lambert law, even though he or she had been able to use it successfully in chemistry class.
Contend with differences in the ways that biologists and chemists use certain words and describe certain phenomena. The language differences surrounding bond energetics, for instance, have historically raised difficulties for students’ understanding: chemists speak of bond dissociation energies, while biologists and biochemists refer to “high-energy bonds” (in molecules such as ATP). A high-energy bond is one that exists at a higher energy level and is therefore relatively unstable toward reaction. However, a bond with high bond dissociation energy represents a particularly stable and unreactive bond. The confusion caused by the language difference can quickly make bonding energetics seem like a very difficult concept!
Complete more science courses in their first year of college by utilizing our January term for one of the courses. If desired, a student can coenroll in the third Ch/Bi course and the second-semester introductory biology course (evolution and diversity) in the Spring term, thus finishing introductory courses in both chemistry and biology during the first year of college.
Ch/Bi was first offered in 2007–2008 to 24 students. In the Fall of 2011, it enrolled a maximum of 56 students (it is limited by the need to run concurrent lab sessions during January term). Assessments of the students exiting the sequence have demonstrated the successful attainment of the goals mentioned above (Abdella et al., 2011). Importantly, though, it seems that an unintended outcome has trumped our original goals: the cohort of students who complete the three semesters of Ch/Bi together find that this learning community becomes a support/study/social group for their entire college careers. Student interest in the program continues to grow. Incoming first-year students now know to ask how they can apply for Ch/Bi.
Neuroscience Program
HHMI funds were also instrumental to the 2000 launching of our interdisciplinary neuroscience program, for which we received start-up funds for new neuroscience faculty and funds to equip a neuroscience teaching laboratory. Since 2000, we have recruited and tenured three neuroscience faculty, two in psychology and one in biology. We have also established a neuroscience concentration (similar to a minor at other institutions) that has grown from four graduates in 2002 to 18 slated to graduate in Spring 2013. Several new courses have been added to the curriculum, including Introduction to Neuroscience, Neuroethology, Neurobiology of Learning and Memory, Neuroscience of Addiction, Neurobiology of Psychopathology, and Advanced Research Methods in Behavioral Neuroscience. Many students also conduct independent research projects with neuroscience faculty and regularly present at regional and national conferences.
In addition to the concentration, a number of students have elected to create an independent major in neuroscience in the past several years under the auspices of our Center for Interdisciplinary Studies. With the guidance of a neuroscience faculty member, each student-created major typically includes courses from biology, psychology, chemistry, statistics, and philosophy.
Physics Initiatives
In 2004, again with the support of HHMI, the physics department developed interdisciplinary course materials for its introductory physics sequence for science majors. The intention was to increase the relevance of the course to its students by drawing on topics related to the biological and chemical sciences. Development took place on two fronts:
Three faculty from physics, biology, and chemistry worked together to develop classroom materials that could exist as “ready-to-use” modules for physics faculty teaching these courses. Resulting materials include modules on biological circuits and molecular spectroscopy.
Two summer student researchers were hired to develop interdisciplinary laboratory experiments. The results include an electrophoresis/circuits lab, a microscope/optics lab, and a positron emission tomography/nuclear radiation lab.
These implementations have proven to be successful and long-lasting, with surveyed students indicating a higher degree of relevance of the physics course to their academic interests.
Biology within the Mathematical Sciences
Members of the mathematics, statistics, and computer science (MSCS) department have worked toward increasing the relevance of their courses to biology students. Recent offerings include Bioinformatics, Mathematics of Biology, and Biostatistics.
Additionally, MSCS and biology faculty developed a seminar course, Exploring Biomath, which has been taught since 2009. This course is organized by a mathematician but is listed as a biology course. It is taught by a group of six faculty members from biology, physics, and MSCS, with each instructor preparing a 2-week unit revolving around an interesting or novel quantitative technique important to biology and ecology. The seminar course exposes students to the primary literature and is open to students who have completed a semester of calculus and an introductory biology course. The success of this course has accelerated the synergy between the two departments.
In Fall 2011, a tenure-track mathematical biologist came onboard in the MSCS department. The new hire organized a Mathbio Working Group that included interested faculty from the MSCS and biology departments. This group has generated a set of initiatives and will be putting their ideas into action in the upcoming years. One goal is to establish a mathematical biology concentration. The existence of a group of faculty having a vested interest in the interdisciplinary area of quantitative biology ensures that continued developmental effort will be invested in this area.
LOOKING BACK
Although there was not a defining moment when we decided to “go interdisciplinary,” several key factors enabled us to move in this direction. For one, it was helpful that, as we created a more interdisciplinary environment, we were always mindful of the importance of rigorous disciplinary grounding and the value of departmental affiliations. Therefore, we were committed from the start to finding the right balance between recognizing the merits of disciplines and departments, while still seeking to be on the leading edge of interdisciplinary ventures.
As we began to develop a shared vision about interdisciplinarity, we were assisted by a change in the administrative structure of the academic program in the late 1990s, one that moved us to a divisional dean approach, in which faculty members serve as administrative leaders for their respective divisions. For the first time, this created a leadership position for a person whose job was to think broadly about the well-being of the division as a whole, a position with the visibility and means to lead a transformation of our approach to science education.
On a practical level, this administrative change fostered the beginning of weekly meetings of the department chairs and associate dean. Over time, this helped create a greater understanding of what was happening across the division, fostered a sense of camaraderie among the divisional leadership, and provided a forum for sharing interdisciplinary ideas and developing strategies for their implementation. Combined with faculty members who were on their own becoming increasingly aware of the emerging emphasis on interdisciplinarity, transformation was, for the most part, both “bottom-up” and “top-down.”
There were definitely bumps along this road related to differing perceptions of importance and appropriate pace and magnitude of change. In the end, transformation occurred through the combined efforts of faculty members who were actively participating in the national debate on changing pedagogical approaches and a leadership team committed to substantive but reasonably paced change. And with that, it just sort of happened: no loud prophetic voices, no administrative demands for immediate change, and no “throwing out the departmental/disciplinary baby with the bathwater.”
We have never known a uniform perspective on interdisciplinarity, and we probably never will. However, we have, to borrow John Gardner's words (1990), achieved a “workable unity” in our quest to respond to the 21st-century call for inquiry-minded students who think outside traditional disciplinary boxes.