The Missing Dimension in Developmental Biology Education
Abstract
Note from the Editor
Points of View (POV) address issues faced by many people within the life science education community. CBE—Life Sciences Education (CBE-LSE) publishes the POV Feature to present two or more opinions published side-by-side on a common topic. We consider POVs to be “Op-Ed” pieces designed to stimulate thought and dialogue on significant educational issues. They are not meant to be exhaustive treatments of a subject.
In this issue, we ask the question, “What are key concepts in developmental biology?” We present three POVs. The first is by CBE-LSE Editor-in-Chief, William Wood, and it is in part based on his experience teaching developmental biology to undergraduates at the University of Colorado, Boulder, including his collaborative experiments in the classroom with Jennifer Knight, the first results of which have been published in CBE-LSE (Knight and Wood, 2005). The second, a partially tongue-in-cheek list of key concepts to convey to students about embryonic development, is by Scott Gilbert (Swarthmore College), author of the leading textbook worldwide for teaching developmental biology, Developmental Biology, 8th ed. (Sinauer Associates, Inc.). The third is by Jeff Hardin (University of Wisconsin–Madison), who has produced Web-based educational materials for teaching developmental biology that are used nationally and internationally for conveying dynamic events during early development (see the WWW feature in this issue by Stark for more details), and who deals with the vexing problem of trying to convey the essential four-dimensional nature of embryonic development to introductory students.
FOUR-DIMENSIONAL THINKING: AN INHERENT CHALLENGE IN DEVELOPMENTAL BIOLOGY
I arrived at the University of Wisconsin–Madison as a young assistant professor in 1991. In those days, teaching a modern course in developmental biology was an exciting proposition. Modern discoveries at the molecular level due to work in invertebrate model organisms were just beginning to be synthesized into coherent “nuggets” that could be passed on to undergraduates, and the pursuit of the molecular basis of the Spemann-Mangold organizer was hot and heavy. Those were heady days indeed. As time passed, however, the challenges of teaching modern developmental biology changed. How could one convey the fruits of the explosion in molecular detail to the modern student (see the accompanying POV by Wood in this issue), while preserving the essential—and intellectually elegant — heritage of classical embryology from the past (as touched on in the POV by Gilbert in this issue). Using girth as a simple guide to the explosion of “facts” in upper-division developmental biology courses, the authors of developmental biology texts initially struggled to deal with this problem as well.1 Of course, these are not problems unique to developmental biologists; my cell biology colleagues lodge very similar complaints when it comes to the core curriculum in their upper-division courses, and at least some cell biology texts have similar struggles with heft (e.g., see the review by Schwarzbauer, 2003;http://www.lifescied.org/cgi/content/full/2/1/16).
The issue of how to convey the essential facts and concepts of developmental biology to undergraduates, given this explosion in knowledge, is clearly important; however, a crucial question remains. Are there other issues that are somewhat unique to teaching developmental biology, as opposed to cell biology, molecular biology, genetics, or biochemistry? One can make the case that an essential feature of developing embryos that is not obviously shared with key topics in these other disciplines, is that the embryo must be constructed over time, and in three dimensions. Because this process, which developmental biologists usually call morphogenesis, occurs in both time and space, it is inherently a four-dimensional (4D) process. This has long been recognized by developmental biology researchers, including those in my own laboratory, who use 4D microscopy in their research to chart the positions of cells as they move to new positions within the embryo (e.g., Thomas et al., 1996; Hardin, 2006). It is this 4D nature of development that allows for new interactions between differentiated parts of the embryo.
FOUR-DIMENSIONAL THINKING IS NOT PART OF THE CURRICULUM
Although 4D thinking has become part of the research repertoire of many developmental biologists, it has yet to make much of an impact on the average undergraduate. There are likely several reasons why this gap persists. First, the processes involved are inherently difficult to grasp at a truly 4D level, even for professional researchers. As a result, as anyone who has struggled to teach the basic features of amphibian gastrulation to uninitiated undergraduates quickly realizes, inculcating a deep understanding of the spatial relationships between parts of the gastrula is one of the most challenging aspects of teaching developmental biology.
Second, as a pragmatic response to the difficulty of learning in four dimensions, it is simply easier to concede the difficulty of the problem, and “solve” this problem by acting as if the embryo is not actually developing in four dimensions. One classic way of doing this in an earlier period was to couple a lecture course in developmental biology to a laboratory course in “embryology,” in which one examined serial sections of embryos at various stages in their development. This approach forces students to develop a three-dimensional (3D) understanding of the embryo by mentally reconstructing such sections, an activity aided by classic atlases of developmental biology (e.g., Schoenwolf, 2007). However, this approach does not usually lead to a 4D understanding of the embryo. This is because one dimension is usually missing in this approach: the transformation of the embryo over time. Moreover, although there are some institutions that still have such courses, they are highly endangered, in the United States at least. As teaching budgets have shrunk, elective laboratory courses have been a convenient target of cuts, particularly at large public universities. In addition, as the emphasis has shifted to molecular approaches in developmental biology, the emphasis of those laboratory courses that remain has shifted in a corresponding direction. As a result, this older method for teaching embryonic structure is disappearing.
Modern computer and animation technology would seem to be a promising avenue to pursue the teaching of the 4D nature of embryonic development. Indeed, I have spent considerable effort over many years to try to provide simple movies and animations as an aid to student learning.2 However, such materials are usually only used to provide visual impact regarding how dynamic development is (thus, they provide the “wow” factor during a lecture). I use them myself this way for the most part. However, video materials have rarely been exploited to aid genuine 4D understanding. It is only when such movies are coupled to more insightful representations of the internal components of embryos as they change over time that such movies will aid 4D thinking regarding the early embryo.
CAN FOUR-DIMENSIONAL THINKING BE LEARNED?
To see why 4D thinking is needed to truly understand animal development, consider one of the most difficult cases commonly covered in an undergraduate developmental biology course: amphibian gastrulation. Gastrulation is a key stage during early animal development, and, as the POV by Gilbert in this issue underscores, it is a truly crucial time in the life history of animals. Largely due to the work of Ray Keller and colleagues, we now have a detailed understanding of how the Xenopus gastrula changes shape in four dimensions (e.g., see review by Keller et al., 2003). This impressive work originated with detailed fate maps of both the interior (deep) cells of the embryo, and its outer (superficial) cells. Although the detailed changes in shape of various regions of the embryo have been well depicted (Figure 1, A and B), it is hard to convey to students how these changes take place dynamically during gastrulation. Because of the difficulty, students are often exposed to a simple two-dimensional (2D) representation of a frog gastrula over time (Figure 1, C and D). Such representations are no doubt a useful starting point, but they are hardly 4D.
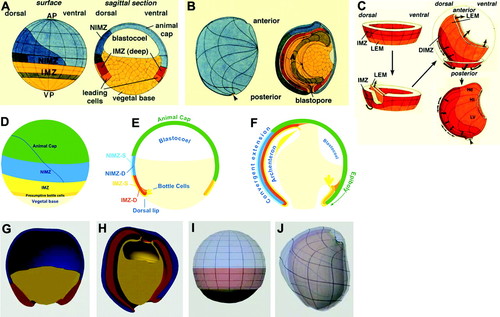
Figure 1. 4D thinking is needed to understand amphibian gastrulation. (A–C) A fully detailed representation of cell movements during gastrulation in Xenopus laevis. Fate maps of the superficial and deep cells of a Xenopus gastrula (adapted from hand drawings made by R. Keller). Dorsal is to the left. (A) Surface and sagittal views of an early gastrula. AP, animal pole; VP, vegetal pole; IMZ, involuting marginal zone; NIMZ, noninvoluting marginal zone. Light blue, presumptive epidermis; dark blue, presumptive neural ectoderm; darkest blue, presumptive floor plate, a type of neural ectoderm; yellow, presumptive endoderm; green, presumptive archenteron roof. The IMZ material moves into the interior, whereas the NIMZ material remains on the surface but changes shape. (B) Late gastrula. A, archenteron. (C) Fate map showing only deep cells. The deep cells form a doughnut-shaped structure around the equator of the embryo, which turns inside-out starting on the dorsal side. The entire structure then extends to cover the entire interior of the embryo, creating an extremely difficult spatial problem for student learning. Orange, leading edge mesoderm; red, deep cells of the involuting marginal zone. (d–f) Simplification of gastrulation into a 2D problem over time. 2D depictions of Xenopus gastrulation over time (from a Flash animation courtesy of D. Gard). (D) Surface view, corresponding to A. Green, animal cap; blue, noninvoluting marginal zone; yellow, involuting marginal zone (IMZ); orange, presumptive bottle cells; tan, vegetal base. (E and F) Sagittal views. (E) NIMZ-S, superficial cells of the noninvoluting marginal zone; NIMZ-D, deep cells of the noninvoluting marginal zone; IMZ-S, superficial cells of the involuting marginal zone; IMZ-D, deep cells of the involuting marginal zone. Material moves into the interior first at the dorsal lip of the blastopore, driven by bottle cells, which constrict. (F) As gastrulation proceeds, the blastocoel is occluded, and the archenteron forms; this is driven by convergent extension of the marginal zone material. (G–I) Two different 3D views of early and late gastrulae (from Flash animations courtesy of Wesleyan University; http://learningobjects.wesleyan.edu/gastrulation/animations.php?ani = 3D). Dorsal is to the left. These animations contain less information than the 2D animation in D–F, but they depict it in a 3D manner over time (i.e., four dimensionally). (G and H) 3D depiction of germ layers. Blue, ectoderm; red, mesoderm; yellow, endoderm. The red material on the left in H has extended more than that on the right. The blastocoel is visible as the cavity in H. (I and J) Partially transparent rendering of ectoderm (light blue) and mesoderm (pink) at early (I) and late gastrula (J) stages. Note that the light blue and pink material on the left extends more dramatically than that on the right, as can be judged by the distortion of the grid lines in J.
Is training in 4D thinking possible at the undergraduate level? Such spatial visualization is an extremely important skill in many fields in science and mathematics. Chemical bond angles, geological structures such as faults and folds, and 3D functional representations are all examples in which spatial thinking is an asset. Spatial visualization is a complex process that involves both visual processing and the construction and manipulation of mental images (Mathewson, 1999). It involves several related mental activities, including the ability to rotate objects about one or more axes (Shepard and Metzler, 1970), the ability to mentally manipulate objects (e.g., by folding them; Ekstrom et al., 1976), and the ability to see through the surface of an object into its interior (“penetrative” thinking; Kali and Orion, 1996).
Despite the importance of spatial visualization in science, mathematics, and engineering, it is not often thoughtfully taught or assessed (Mathewson, 1999). Moreover, longitudinal studies suggest that failure to train students in this area can lead to their abandoning certain fields entirely (e.g., Shea et al., 2001). Fortunately, although students have differing aptitudes for such spatial thinking, psychological research suggests that training in spatial thinking is possible for most students (Lord, 1985), and that such training is effective irrespective of gender (Sorby, 2001; Levine et al., 2005; Feng et al., 2007).
With regard to developmental biology in particular, the article by Lu and colleagues in this issue of CBE-LSE shows that students can learn the rudiments of 4D thinking by exposure to raw 4D data sets of embryos if the embryos are inherently simple in organization, as Caenorhabditis elegans early embryos are. Even here, however, as the structure of the embryo becomes progressively more complicated, a via media is necessary, in which only the salient features of the development of specific structures are highlighted amid the complexity of the entire embryo. Clearly, an intermediate sort of representation, in which salient features of the 4D embryo are depicted, is what is needed to help students grasp the key features of gastrulation.
Computer-aided representations may be particularly useful in this regard. Studies have shown that computer games can be useful in a general sense to train students to think spatially (Feng et al., 2007). With regard to the specific problem of understanding gastrulation, computer rendering may be particularly valuable. For example, it is possible to depict structures of an otherwise opaque embryo with varying degrees of transparency to aid penetrative thinking skills (Figure 1, E and F), and it is possible to extract particular features in a 4D representation to highlight important architectural features of the embryo (Figure 1, G and H). Although the examples shown in Figure 1, E–H, are derived from static orientations, the technology already exists to depict embryos on the computer as true 3D objects in 4D space. What is needed is the application of instructional materials development resources toward the production of such models. If such models become widely available, it should be possible to reclaim all four dimensions of the embryo in the undergraduate developmental biology curriculum.
FOOTNOTES
This can actually be demonstrated empirically in the case of the classic text in the field, Developmental Biology, by Scott F. Gilbert (Sinauer Associates, Inc.). The thickness of various editions is as follows (publication date, thickness in centimeters, and page count are shown in parentheses): 1st (1985, 3.7 cm, 726 pp.), 2nd (1988, 3.6 cm, 843 pp.), 3rd (1991, 3.6 cm, 891pp.); 4th (1994, 3.7 cm, 894 pp.), 5th (1997, 3.9 cm, 958 pp.), 6th (2000, 2.9 cm, 749 pp.), 7th (2003, 3.3 cm, 838 pp.), and 8th (2006, 3.3 cm, 817 pp.). There was clearly a period in the late 1990s during which the new knowledge had to be consolidated.
See http://worms.zoology.wisc.edu/embryo_main/embryology_main.html for the old site, covering echinoderms and amphibians, and the new, higher bandwidth site, which has thus far only been updated to include echinoderms, at http://worms.zoology.wisc.edu/dd2/.
ACKNOWLEDGMENTS
I thank Ray Keller (Department of Biology, University of Virginia) for the use of classic drawings, David Gard (Department of Biology, University of Utah) for the use of Flash animations of gastrulation, and the instructional materials development team at Wesleyan University (http://learningobjects.wesleyan.edu/) for bringing animations of gastrulation to my attention. I also thank Ryan King for helpful discussions regarding how to assess 3D and 4D thinking. I especially thank Sarah Titus (Department of Geology, Carelton College), Eric Horsma, and Basil Tickoff (Department of Geology and Geophysics, University of Wisconsin–Madison) for helpful interactions about penetrative thinking, why geologists and developmental biologists face similar 4D problems, and how to think about their assessment.