Building Authentic Science Experiences: Students’ Perceptions of Sequential Course-Based Undergraduate Research
Abstract
Course-based Undergraduate Research Experiences (CUREs) are attractive solutions for scaling undergraduate research experiences at primarily undergraduate teaching institutions, where resources for faculty research activities can be limited. The Sustainable Interdisciplinary Research to Inspire Undergraduate Success (SIRIUS) project is a unique program that integrates CUREs, coordinated around a local real-world problem, throughout a biology department’s curricula. The CUREs are scaffolded to provide all biology majors with multiple opportunities to engage in scientific investigations as they advance through introductory, intermediate, and advanced courses. In this mixed methods, cross-sectional study, we explore students’ perceptions of the authenticity of their experiences as they progress through the SIRIUS CUREs. Triangulated data collected from two instruments indicated that students in advanced courses recognized more involvement in research activities and perceived greater authenticity in the science they were performing compared with introductory and intermediate students. Intermediate and advanced students perceived more opportunities for independence; however, experiences with failure and the influence these experiences had on the perceptions of authenticity was primarily observed with advanced students. This study contributes to the growing literature on CUREs with a focus on students from a primarily undergraduate institution with multiple minority-serving designations.
INTRODUCTION
Only half of all students that enter college declaring a Science, Technology, Engineering, or Mathematics (STEM) major graduate with that degree (Chen & Ho, 2012), and this drops to one-fourth for students identifying as Persons Excluded because of their Ethnicity or Race (PEERs; Asai, 2020). One strategy for retaining undergraduate students in STEM disciplines is to provide them with opportunities to participate in experiential learning (e.g., internships, service learning, research). Undergraduate Research Experiences (UREs) have been shown to benefit students in a variety of ways, including enhancing their scientific thinking, science identity, self-efficacy, and in some cases, retention and graduation in their chosen field (Nagda et al., 1998; Seymour et al., 2004; Hunter et al., 2007; Lopatto, 2007; Kuh, 2008; Jones et al., 2010; Eagan et al., 2013; Linn et al., 2015; Robnett et al., 2015).
While UREs offer valuable experiences, access to these opportunities can be limited and exclusive. Student participation in UREs is often dependent on faculty time and resources, students’ awareness of research opportunities and benefits, and students’ knowledge and confidence in pursuing research positions (Carlone & Johnson, 2007; Bangera & Brownell, 2014; Munawar, 2015; Cooper et al., 2021). In addition to those barriers, UREs are often unpaid and require extra time outside of class, posing additional inequities, especially for those with socioeconomic constraints and nonacademic demands (Bangera & Brownell, 2014). Course-based Undergraduate Research Experiences (CUREs) represent opportunities for students to participate in research as part of their course curricula (Buchanan & Fisher, 2022). Replacing a prescriptive “cookbook” lab with an authentic investigation (e.g., exploring bacterial diversity in samples collected at different times during a wetlands restoration project) can eliminate many of the barriers students face when accessing faculty-mentored UREs.
Our study used the CURE framework as a theoretical lens to construct our research questions, study design, and analysis strategy. Initially described by Lopatto and colleagues (2008) as “Classroom Undergraduate Research Experiences,” CUREs were further defined by the Course-based Undergraduate Research Experience Network (CUREnet). The latter was established in 2012 to support classroom instruction that integrated five curricular elements: 1) Scientific Practices, 2) Discovery, 3) Relevance, 4) Collaboration and 5) Iteration (Auchincloss et al., 2014; CUREnet). As defined by Auchincloss and colleagues (2014), scientific practices include learning and practicing basic skills/techniques used by scientists (e.g., pipetting, polymerase chain reaction, lab notebook maintenance), as well as applying the process of science (e.g., hypothesis generation, experimental design, data collection/analysis). Discovery refers to the generation of novel data and requires outcomes of student work to be unknown to the students and instructors. Relevance requires that student work is important beyond the classroom (e.g., to the scientific community, local community, or other stakeholders). Collaboration refers to engagement in meaningful research activities with others, such as group experimentation, sharing and discussing ideas, and peer review. Iteration can involve expanding on the work of others or performing an experiment multiple times to troubleshoot issues that arise. The definition of a CURE, and ideas concerning the degree to which each component is emphasized, continues to evolve as the pedagogical strategy is more widely implemented and tested; yet the features described above remain essential to the curricular design.
The benefits of CUREs have been shown to mirror those of UREs (Lopatto et al., 2008). A growing literature cites enhanced self-efficacy, career clarification, and improved retention and graduation rates in students participating in a variety of CURE models (Brownell et al., 2012, 2015; Corwin, Graham et al., 2015; CUREnet; Olimpo et al., 2016; Rodenbusch et al., 2016). For instance, the Freshman Research Initiative, which includes a series of three CUREs for first-year biology students at the University of Texas, Austin, observed an increase in the six-year graduation rate for students who participated (Rodenbusch et al., 2016). Other studies have reported increased content knowledge and scientific thinking (Brownell et al., 2012, 2015), as well as an increased sense of project ownership (Corwin et al., 2018; Cooper et al., 2019), which in turn, has been linked to other psychosocial and attitudinal states that may influence retention (Hanauer et al., 2016). Whereas early investigations predominantly compared CUREs to other laboratory experiences, recent studies have sought to link discrete components or aspects of CUREs to specific student outcomes (Gin et al., 2018; Cooper et al., 2019; Wiggins et al., 2021).
Few studies have explored students’ perceptions of science or research authenticity in their CUREs, and our study aims to expand on this literature. While we primarily draw on the CURE framework established by Auchincloss et al., (2014) for our definition of research authenticity, we acknowledge the rich literature and variety of definitions that have been applied to the term “authentic” as it is used in science education. Rowland and colleagues (2016) present 26 definitions of “authentic science/research” from a literature review they conducted to construct a framework for the evaluation of their authentic large-scale URE (ALURE) curricula. The authors designed their laboratory courses to emphasize the “real-world” tasks included in the definition of authenticity posed by Jonassen and Rohrer-Murphy (1999), the novel discovery emphasized by Weaver and colleagues (2008), and the concept of flexibility to incorporate product or process-based activities promoted by Spell et al., (2014). However, they also emphasize the importance of collecting students’ perceptions of authenticity to assess the "delivered" curriculum. Furthermore, it has been suggested that authenticity is a dynamic quality of science that relies upon negotiation among those doing science; thus, students working on a project must agree that their project is meaningful, and they must understand for themselves that their work is authentic (Rahm et al., 2003). In a study of high-school students engaging in computer-based and wet lab CUREs, students’ deeply held stereotypes of what scientists do may have influenced them to perceive the wet lab module as more authentic than the database project, despite students recognizing more research tasks performed in the database activity (Munn et al., 2017).
The Laboratory Course Assessment Survey (LCAS) indirectly arrives at student ideas of CURE authenticity by examining the perceptions that students have about participation in activities related to collaboration, novel discovery with broad relevance, and iteration (four of the five CURE curricular components; Corwin, Runyon et al., 2015). Goodwin and colleagues (2021) supplemented the LCAS with an open-ended question that more directly assessed students’ views of authenticity in their comparison of an inquiry-based and CURE laboratory. They found that both student populations recognized they were participating in research-based activities; however, CURE students reported more engagement. Furthermore, by asking students if they thought their laboratory experiences were “real research,” they were able to discern that the CURE curricular elements of scientific practices, discovery with relevance, and iteration, as well as opportunities to fail, were important factors in students’ perceptions of authenticity.
Context of the current study
The Sustainable Interdisciplinary Research to Inspire Undergraduate Success (SIRIUS) project represents one of the first CURE models to be coordinated and implemented extensively throughout a department. The SIRIUS Project adopts a structure in which a series of CUREs are coordinated across a single department’s curricula to address a problem of interest and concern to the local community (i.e., a place-based model; McDonald & Landerholm, 2018; McDonald et al., 2019). SIRIUS was first conceived of, and implemented by, faculty in the Department of Biological Sciences at a minority-serving, comprehensive teaching institution in the western United States. During a multi-year Faculty Learning Community (FLC), CUREs and CURE modules (or mini-CUREs) were designed for 12 courses spanning introductory through capstone laboratories with research activities focused on the health of, and human impacts on, the American River and its surrounding ecosystem.
The overarching goals of the SIRIUS Project are to 1) provide research opportunities to all biology students at the institution, 2) develop a scientific community where students, faculty, and staff work collaboratively on a local problem, and 3) scaffold curricula to allow students multiple opportunities to build their research skills and identity (McDonald & Landerholm, 2018; McDonald et al., 2019). At the time of graduation, biology majors have generally participated in four to eight SIRIUS Project courses, thus performing research across multiple sub-disciplines of biology. As of 2020, over 5000 students participated in SIRIUS courses and a comprehensive evaluation plan to study the impacts of the program has assessed a variety of student and faculty outcomes (McDonald et al., 2019; Martin et al., 2021).
This widespread implementation allowed us a unique opportunity to observe how student perceptions of whether they were doing real (“authentic”) science differed after completing CUREs in courses at varying levels (introductory to advanced), as well as compare the rationales for their perceptions. While the study was cross-sectional, and therefore changes in individual students or cohorts could not be tracked, students in the advanced classes would have had several CURE experiences before taking the advanced class. Thus, at least some observed outcomes could be due to the cumulative effect of experiencing multiple related CUREs. Here, we present data from an examination of the perceptions students hold about the authenticity of their lab experiences at three timepoints (introductory, intermediate, and advanced). Specifically, we address the following research questions:
Do students recognize participating in research-based activities in their laboratory curricula?
Do students perceive their laboratory experiences to represent authentic science? Why or why not?
Do perceptions and rationales of authentic science differ between students enrolled in introductory, intermediate, and advanced CUREs or across different demographic groups?
METHODS
SIRIUS courses
The courses included in this study spanned the curricula and contributed to the requirements or electives of all concentrations offered by the Department of Biological Science (i.e., General BA and BS; Biomedical Sciences; Cell and Molecular Biology; Clinical Laboratory Sciences; Ecology, Evolution and Conservation; Forensic Biology and Microbiology). The research projects differed between individual courses (Table 1), but all curricula related to the health of and/or human impacts on the American River, which runs through campus and is listed as Impaired under Section 303(d) of the federal Clean Water Act of 1972. As described in the following section, SIRIUS courses were categorized according to introductory, intermediate, and advanced levels. All courses included in the SIRIUS Project have lecture and lab components, and CURE curricula were integrated into laboratory sections (and in the case of BIO1, a discussion section). Only the nine SIRIUS laboratory courses that met the definition of a CURE were evaluated in this study. We used syllabi, course materials, and discussions with faculty to ensure that their CURE engaged students in scientific practices, involved collaboration and iteration, and addressed a novel question with relevance outside their specific course (Fajardo, 2022).
Course level | Course title | Number of students per semester | Research topic |
---|---|---|---|
Introductory | BIO 1: Biodiversity, ecology and evolution | ∼384 | Fertilizer effects on American River macroinvertebrates |
BIO 2: Cells, molecules and genes | ∼142 | Urban run-off effects on American River soil bacteria | |
Intermediate | BIO 139: General microbiology | ∼142 | Identification of fecal coliform bacteria from the American River |
BIO 160: General ecology | ∼72 | Ecology of American River tree root fungi | |
Advanced | BIO 127: Developmental biology | ∼48 students per year (Fall semesters only) | Human-derived toxin effects on population dynamics of C. elegans nematodes |
BIO 128: Plant physiology | ∼24 students per year (Spring semesters only) | Effects of restoration adjacent to the American River on mycorrhizal fungi diversity | |
BIO 145: Diversity of microorganisms | ∼16 students per year (Spring semesters only) | Identification and sequencing of American River antibiotic-producing bacteria (Tiny Earth) | |
BIO 180: Advanced molecular biology | ∼32 students per year (Spring semesters only) | Genetic engineering of American River soil bacterium Acinetobacter baylyi | |
BIO 187: Advanced cell biology | ∼16 students per year (Spring semesters only) | Analysis of the effects of toxins on the survival of mammalian cell cultures |
CURE levels
SIRIUS Project CURE courses were assigned to introductory, intermediate, and advanced levels (Table 1) based on a combination of three parameters: 1) the year in a student’s undergraduate career the courses are typically taken (as first-year student, Sophomore, Junior, Senior or Graduating Senior), 2) the number of Biology and Chemistry prerequisites required by the course, and 3) the nature of the curricula; in particular, the length of research project and the inclusion of central versus peripheral tasks (Table 2). Central tasks are fundamental to the scientific method and may involve developing a research question and/or experimental design (Corwin, Graham et al., 2015; Dolan & Weaver, 2021). Peripheral tasks are those that a novice could engage in to learn and contribute to an experiment and are also tasks that allow for mistakes and repetition, such as data collection and analysis (Lave & Wenger, 1991; Corwin, Graham et al., 2015; Dolan & Weaver, 2021). The introductory courses are the first biology courses taken by majors (and some nonmajors) and have few or no prerequisites. Students in introductory courses learned and practiced peripheral tasks (e.g., calculations and pipetting) at the beginning of the semester, then proceeded to more advanced peripheral tasks (e.g., collecting and analyzing data) in the next stage of their project; however, the research questions were defined for them. In one of the introductory courses, students performed central tasks when revising experimental designs for a second iteration of experiments. Introductory courses are prerequisites for intermediate courses, which are typically taken in years three and four. Intermediate courses built on skills learned at the introductory level; however, activities included more central tasks, such as making choices regarding strategies and tests that should be performed given a specific experimental objective. Advanced courses have the most prerequisites and are often composed of students who have senior class status and are nearing graduation. Advanced courses incorporated higher levels of inquiry (Buck et al., 2008), with CURE projects that were typically longer and allowed students more autonomy in decision making. Advanced CURE students engaged in more central tasks compared with the other levels, such as generating research questions, developing proposals, and experiments.
Level | Course | Year taken | Cumulative Prerequisites (BIO and CHEM) | Length of CURE | Instructor type | FLC Participant |
---|---|---|---|---|---|---|
Introductory | Bio 1 | First Year/So, non-majors | none | 30% of semester | TT, LTL, L, GTA | TT, GTAs |
Bio 2 | So/Jr | 2 | 75% semester, with skills building included | TT, LTL, L, GTAs | TT, L | |
Intermediate | Bio 139 | Jr/Sr | 6 | 30% actual experiment but prior weeks for skills development necessary | TT, LTL, L | TT, LTL |
Bio160 | Jr/Sr | 5 | Full semester | TT, LTL, L | TT, LTL | |
Advanced | Bio127 | Sr/Graduating Sr | 7 | 50% for research question, but prior weeks for skills development | TT | TT |
Bio128 | Sr/Graduating Sr | 4 | Full semester | TT | TT | |
Bio145 | Graduating Sr | 7 | Full semester | TT | TT | |
Bio180 | Graduating Sr | 6 | Full semester | TT | TT | |
Bio187 | Graduating Sr | 6 | Full semester | TT | TT |
In addition to curricular differences, faculty type and involvement in the SIRIUS professional development activities differed between levels (Table 2). In advanced classes, smaller enrollments allowed single instructors to teach all aspects of the course. These instructors all participated in the SIRIUS professional development. In the larger introductory and intermediate classes, faculty participating in SIRIUS designed the CUREs, but had less control over the implementation of the curricula in the multiple laboratory sections taught by lecturers. All instructors teaching CUREs at the introductory and intermediate levels were aware of the project, but not all had the same degree of training or level of investment.
Assessment instruments
Laboratory course assessment survey (LCAS).
The 17-item Laboratory Course Assessment Survey (LCAS) was used to measure students’ perceptions of laboratory course curricula across the three CURE levels for three independent constructs: Collaboration, Discovery/Relevance, and Iteration (Corwin, Graham et al., 2015, Supplemental 1). The Collaboration section of the LCAS asked students how often they engaged in six collaborative activities with possible total scores ranging from 6-30. Example activities in the Collaboration section are “discussing elements of their investigation with classmates or instructors,” “helping other students collect or analyze data,” and “providing constructive criticism to classmates.” The Discovery/Relevance construct of the LCAS consisted of five items that prompted students to rank their level of agreement that they participated in activities such as “conducting an investigation to find something previously unknown,” and “developing new arguments based on data.” Possible total scores in this section ranged from 5 to 30. The Iteration section asked students to indicate their level of perceived agreement that they participated in six activities including, “revising or repeating work to account for errors or fix problems,” “having time to share and compare data with other students,” and “having time to revise drafts of papers or presentations about my investigation based on feedback.” Possible total scores in the Iteration section ranged from 6 to 36.
Authenticity Questions.
Along with the LCAS, we posed two free-response questions to gather students’ ideas about the nature of science and their participation in the process of science (Supplementary 1 and 2). Using a phenomenological approach to understand the CURE experiences from the participants’ perspective (Easterby-Smith et al., 2012), we asked students the questions: “Did you feel like you were doing ‘real science’ in your lab course? Why or Why not?”
Study population and data collection
This study surveyed students enrolled in SIRIUS Project courses at a primarily undergraduate serving institution in the western United States (Table 1) under approved institutional review board protocol #13-14-148. All students enrolled in SIRIUS courses during semesters under evaluation (Fall 2015–Spring 2019) were recruited by their instructors during class to participate in surveys (Table 3). A small number of students appeared in the overall dataset more than once, as they participated in multiple SIRIUS courses during the evaluation timeframe. Survey items were specific to the courses students participated in, and thus were included in the data set.
Assessment instrument | Level | Course | Semester of data collection |
---|---|---|---|
Introductory | Bio 1 | F15, S16, S17 | |
Bio 2 | F15, S16, S17 | ||
1. Laboratory Course Assessment Survey (LCAS) | Intermediate | Bio 139 | F16, S17 |
Bio 160 | F16, S17 | ||
2. Authenticity Question | Advanced | Bio 127 | F16 |
Bio 128 | S17 | ||
Bio 145 | S18 | ||
Bio 180 | F18 | ||
Bio 187 | S18, S19 |
The assessment instruments were administered as post-CURE surveys at the end of each semester under evaluation (Table 3). Surveys conducted in early semesters (2015–2016) were administered in class on paper. Surveys conducted in subsequent semesters (2017–2019) were administered outside of class using the Campus Labs online survey tool.
Data analysis
Demographic data.
Demographic data were obtained from the university’s Office of Institutional Research and variables were summarized using descriptive statistics. Because our analyses involve comparisons across levels, χ2 tests of independence were used to examine the composition of students in each CURE level according to the following demographic groups: gender (Female, Male), race/ethnicity (Asian; Persons Excluded due to Ethnicity or Race, which included Latinx, Black, Native American, and Pacific Islander; White), Pell Grant eligibility status (Pell Grant eligible, Non–Pell Grant eligible) and transfer status (Transfer, Nontransfer). At the time of data collection, the office of institutional research only offered two options for “gender identification” (male and female). While students self-reported this information on admission documents, the limited selection options mean we were unlikely to capture the true gender diversity of our study participants. Also, the term “transfer” in this study refers to any college student transferring from another institution (community college or four-year) to our institution; however, the vast majority of transfer students at our institution (85% or more) are from community colleges.
Quantitative analysis of Laboratory Course Assessment Survey (LCAS).
Student responses for items in each construct (Collaboration, Discovery/Relevance, and Iteration) were examined and eliminated if they met one of three criteria: at least one blank item, at least one response of “I don’t know,” or at least one response of “I prefer not to respond.” The scale for the Collaboration construct was flipped before analysis to be more consistent with the other two constructs. Counts from items in each construct were summed to provide a single score for each student. The LCAS has been validated by prior studies across large undergraduate student populations (Corwin, Runyon et al., 2015; Corwin et al., 2018; Cooper et al., 2019; Goodwin et al., 2021). On our student population, Confirmatory Factor Analysis indicated reasonable fit (CFI - 93.6, TLI - 92.5, RMSEA - 0.066) reasonable fit is defined by CFI and TLI > 90.0 and RMSEA between 0.08 and 0.05 (Bentler, 1990; Byrne, 1994; Fan et al., 1999). Cronbach’s alpha internal consistency reliabilities for each construct Collaboration (α = 0.86), Discovery/Relevance (α = 0.85), and Iteration (α = 0.89), were above the accepted cut off (α = 0.70) (Nunnally, 1978). All statistical tests were performed in R v. 4.0.3 (R Core Team, 2021) using the following packages: psych (Revelle, 2022), ltm (Rizopoulos, 2006), lavaan (Rosseel, 2012), dunn.test (Dinno, 2017), and ggplot2 (Wickham, 2016).
To determine if we could combine courses into introductory, intermediate, and advanced levels for LCAS analyses, we compared courses within each level using ANOVA. We found no significant differences between mean scores in courses within the same level, thus the courses were combined into the three categories for further analysis. Preliminary analyses also indicated nonnormality and nonhomogenous variances. Therefore, nonparametric Kruskal-Wallis tests were used to compare median LCAS scores across levels (introductory, intermediate, and advanced) for each construct. Post hoc Dunn tests with the Benjamini-Hochberg adjustment were used to determine which levels were significantly different from each other (Dinno, 2015).
Qualitative analysis of Authenticity Question responses.
Student excerpts (n = 1,252) from the Authenticity Question were managed and analyzed using Dedoose version 9.0.17 (Dedoose, 2021) and Microsoft Excel (Microsoft Corporation, 2018). A preliminary version of the codebook was developed for a subset of the data by one of the authors (K.M.) and a former researcher. In the preliminary version, analysis of the responses identified: 1) a priori codes that directly represented the CURE curricular elements drawn from the CURE framework (i.e., Scientific Practices, Discovery, Relevance, Collaboration, and Iteration), and 2) emergent codes, which were reoccurring words, phrases, and ideas in student responses. The first codebook, amended by three researchers (B.V., E.P., and H.F.), was used for analysis of a larger data set for this study (Supplementary 3). To develop the new codebook, descriptive coding methods were used to define emergent codes that summarized, in one or two words, the main ideas from students’ responses (Saldana, 2021). The codebook was further adapted by adding, revising, and removing codes as necessary during the iterative coding process, which used an additional subset of the data. For this study, two or more researchers independently applied codes to all student excerpts. Initial percent agreement was 75.7% or greater, and all coding disagreements were resolved by discussion to reach consensus. Representative excerpts were selected to illustrate code usage across introductory, intermediate, and advanced levels. A priori and emergent code frequencies were evaluated for differences across demographic groups (gender, race/ethnicity, Pell Grant eligibility status, and transfer status). Descriptive statistics were used to summarize frequency of Yes/No responses and rationales for Yes/No responses (rationale codes).
RESULTS
Demographic characteristics of students across course levels
From Fall 2015 to Spring 2019, 1115 unique students out of 1252 total student participants completed the surveys, with a majority identifying as female (59.8%), students of color (61.3%), and eligible for Pell grants (53.1%). Demographic data were analyzed to determine the student composition of each SIRIUS CURE level (Table 4). Transfer status was the only factor that differed across the course levels (p < 0.001), with significantly fewer transfer students in the introductory courses than the intermediate and advanced levels. This is due to most transfer students completing their introductory coursework at community colleges.
Demographics | Introductory n = 746% | Intermediate n = 406% | Advanced n = 100% |
---|---|---|---|
Gender | |||
Female | 60.7 | 57.9 | 60.0 |
Male | 37.8 | 39.7 | 39.0 |
Unknown | 1.3 | 2.5 | 1.0 |
Race/Ethnicity | |||
Asian | 29.4 | 32.0 | 26.0 |
PEERs | 33.8 | 27.1 | 28.0 |
White | 26.7 | 28.8 | 27.0 |
Other | 6.8 | 8.1 | 14.0 |
Unknown | 3.4 | 3.9 | 5.0 |
Pell status | |||
Pell eligible | 54.4 | 50.0 | 56.0 |
Non-Pell eligible | 43.2 | 46.3 | 42.0 |
Unknown | 2.4 | 3.7 | 2.0 |
Transfer status* | |||
Transfer | 23.9 | 54.7 | 52.0 |
Nontransfer | 74.7 | 42.6 | 46.0 |
Unknown | 1.5 | 2.7 | 2.0 |
Student perceptions of collaboration, discovery/relevance, and iteration
The Laboratory Course Assessment Survey (LCAS) was used to measure students’ perceived participation in activities related to Collaboration, Discovery/Relevance, and Iteration in their SIRIUS CURE courses. For all constructs, higher scores indicated a higher level of perceived participation. Kruskal-Wallis tests indicated significant differences between course levels for each construct (Collaboration, Discovery/Relevance, and Iteration; Table 5).
Construct | Score Range | Introductory | Intermediate | Advanced | Kruskal–Wallis | |||||
---|---|---|---|---|---|---|---|---|---|---|
Median | IQR | Median | IQR | Median | IQR | p | h | df | ||
Collaboration | 6–30 | 27 | 23–30 | 27 | 22–30 | 30 | 28–30 | 0 < 0.001 | 37.5 | 2 |
Discovery/Relevance | 5–30 | 24 | 21–26 | 23 | 19–26 | 28 | 26–30 | 0 < 0.001 | 103 | 2 |
Iteration | 6–36 | 28 | 24–31 | 26 | 20–30 | 33 | 30–36 | 0 < 0.001 | 114 | 2 |
Response rates for the LCAS portion of the survey were 63.6% for introductory, 72.5% for intermediate, and 66.5% for advanced students. Students in all CURE levels had relatively high Collaboration scores (Figure 1); thus, they reported high levels of participation in activities such as group work and providing feedback to peers. Post hoc Dunn tests indicated advanced students reported participating in collaborative activities more often than introductory and intermediate students (p < 0.001). Although the medians are the same between introductory and intermediate groups, the two groups were statistically different (p < 0.05).
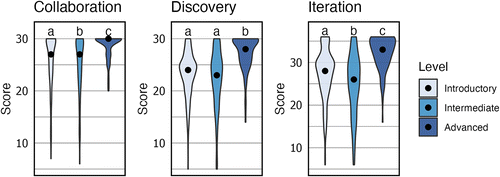
FIGURE 1. Comparisons of LCAS Scores Between CURE Levels. Each construct had a different number of participants due to the exclusion criteria used to prepare data for analysis. Collaboration LCAS scores from CURE course levels: introductory (n = 579), intermediate (n = 342), and advanced (n = 95). Discovery/Relevance scores from CURE course levels: introductory (n = 627), intermediate (n = 337), and advanced (n = 105). Iteration scores from CURE course levels: introductory (n = 652), intermediate (n = 326), and advanced (n = 103). Black dots represent medians. Levels with the same letter are not significantly different (p > 0.05).
The Discovery/Relevance construct of the LCAS prompted students to rank their level of agreement that they participated in activities related to novel discovery with broad relevance. Advanced students had the highest Discovery/Relevance scores rating participation in these activities more often than introductory and intermediate students (p < 0.001; Figure 1).
Differences were reported across all course levels for laboratory activities related to Iteration, such as troubleshooting, repeating and revising work. Advanced students had higher Iteration scores than introductory students, who had higher scores than intermediate students, and post hoc Dunn tests indicated all scores were significantly different from one another (p < 0.001; Figure 1). Overall, the LCAS data indicated that students in every level recognized participating in research-based activities, with advanced students perceiving the most participation with the least score variation (Figure 1 and Table 5).
Student perceptions of science authenticity
A total of 1252 students responded to the survey question “Did you feel like you were doing ‘real science’ in your lab course? Why or why not?” Responses were collected from 746 introductory, 406 intermediate, and 100 advanced students. Response rates for the open-ended authenticity questions were 61.2% for introductory, 79.8% for intermediate, and 63.3% for advanced students. Data collected from the first question, coded as Yes, No or Maybe, indicated the majority of students perceived their laboratory experiences to be “real science.” Eighty-seven percent of students across all levels combined responded positively (i.e., Yes), and as course level increased, the proportion agreeing to the statement increased, with nearly all (98%) of advanced students indicating their laboratory experiences represented “real science.” Advanced students were also more decisive in their responses, as evidenced by the lack of Maybe/Unclear responses (Table 6). The second question, “Why or why not?” offered students the opportunity to provide the reason for their perceptions of authenticity or inauthenticity. Each student excerpt was first coded with the a priori CURE curricular elements, as in the following example from an advanced student whose response included activities or ideas related to Relevance, Original/Discovery, and Iteration.
Responses | Introductory n = 746% (n) | Intermediate n = 406% (n) | Advanced n = 100% (n) |
---|---|---|---|
Yes | 85.0 (634) | 89.4 (363) | 98.0 (98) |
No | 6.8 (51) | 6.2 (25) | 2.0 (2) |
Maybe and Unclear | 8.3 (61) | 4.4 (18) | 0.0 (0) |
“Yes. Because we were working with genes in a system that had not been tested before and investigating a topic that needs more research on. Our research can be used by other scientists in future work to continue understanding gene amplification. Also, the outcome was not known so we were doing novel research.”
Quantifying the CURE curricular elements revealed that the responses from advanced students contained more CURE curricular elements (per excerpt) than responses from introductory and intermediate students (Table 7). The majority of students across all levels mentioned one CURE curricular element in their responses; however, the percentage of students within each level mentioning two to four CURE curricular elements increased with course level. Across all CURE levels, participation in Scientific Practices (>59%, Figure 2) was cited most often as the reason students perceived their lab experiences to be authentic, while Collaboration was mentioned the least (≤5%). Advanced students cited Relevance, Original/Discovery, and Iteration more often than introductory and intermediate students, who reported these reasons at similar frequencies (Figure 2). After Scientific Practices, advanced students mentioned Original/Discovery most often, followed by Iteration, and then Relevance. Intermediate and introductory students both mentioned Iteration as their rationale least often with Relevance cited slightly more often than Original/Discovery.
# Of CURE curricular elements per excerpt | Introductory n = 746% (n) | Intermediate n = 406% (n) | Advanced n = 100% (n) |
---|---|---|---|
0 | 22.1 (165) | 22.9 (93) | 10.0 (10) |
1 | 61.7 (460) | 59.1 (240) | 45.0 (45) |
2 | 14.6 (109) | 16.0 (65) | 33.0 (33) |
3 | 1.5 (11) | 2.0 (8) | 11.0 (11) |
4 | 0.1 (1) | 0.0 (0) | 1.0 (1) |
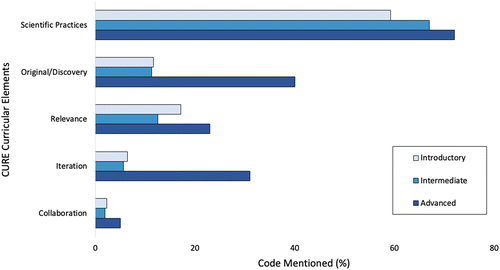
FIGURE 2. CURE curricular elements from authenticity question compared across levels. CURE curricular elements (a priori rationales) across introductory (n = 746), intermediate (n = 406), and advanced (n = 100) students.
Scientific Practices was cited most often by students at all course levels, and due to the broad nature of the code, we performed additional open, descriptive coding to disaggregate it into the following (emergent) sub-codes: Techniques, Answering Questions, Communication, Analysis, Hypothesis Creation, and Fieldwork. Performing various techniques was the most frequently discussed subcode (87.2%) of Scientific Practices across all levels (Figure 3). Advanced students mentioned Answering Questions and Communication more often than students at the other levels; however, all codes other than Techniques were reported infrequently by comparison.
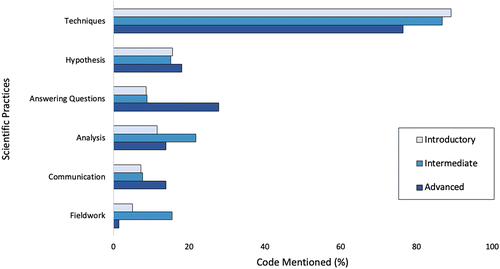
FIGURE 3. Scientific practices disaggregated and compared across levels. Subcodes nested under scientific practices compared across introductory (n = 442), intermediate (n = 272), and advanced (n = 72) students.
Additional emergent codes, identified through inductive open coding, provided further insight into student perceptions of their laboratory experiences. While all emergent codes were cited less frequently than the CURE curricular elements, advanced students referred to Independence (17.0%), and Resilience/Failure (14.0%) more often than introductory and intermediate students (Figure 4), and intermediate students mentioned experiencing Independence (13.5%) and Resilience and Failure (3.20%) more often than introductory students (4.29%, 0.27% respectively), which may point to increased rigor across the scaffolded laboratory curricula.
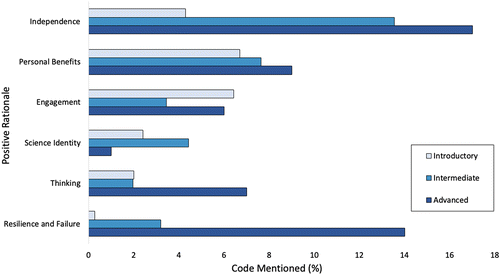
FIGURE 4. Emergent positive rationales compared across levels. Codes are compared across introductory (n = 746), intermediate (n = 406), and advanced (n = 100) students.
Five codes emerged from responses of students who did not think their labs represented “real science”: Limited, Cookbook, No Structure, No Engagement and No Relevance. Although these negative emergent codes were cited infrequently (<9%) by students in all levels, introductory students were more likely to question their laboratory curricula’s inauthenticity for these reasons (Figure 5). The most common code for this group was “Limited,” which we applied when students mentioned feeling their experience was lacking something, such as time to perform a certain task, involvement in study design, or access to materials necessary for experimentation. Limited and Cookbook were the only two negative codes to emerge from advanced student responses, whereas a few introductory and intermediate students also indicated that their labs lacked structure or relevance or were not engaging. Example quotes for the a priori CURE curricular element codes and the most frequently reported emergent codes are provided in Table 8. These quotes are representative of the code descriptions and serve to illustrate the differences in student responses across CURE levels.
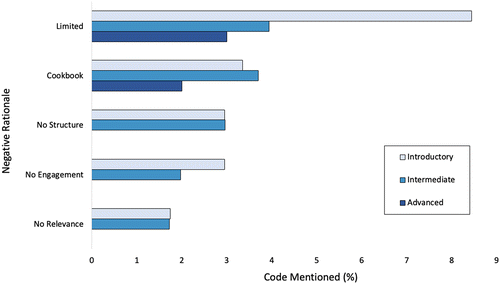
FIGURE 5. Negative rationales compared across levels. Codes are compared across introductory (n = 746), intermediate (n = 406), and advanced (n = 100) students.
Authenticity code | Definition | Introductory quote | Intermediate quote | Advanced quote |
---|---|---|---|---|
Scientific practices | Using the scientific method, including skills or tools used to do so | Yes, it was my first time doing an experiment on site rather than just using the samples and solutions given the lab room. | Yes. We took samples from the American River and recorded our data. | Yes. I collected the sample and processed everything through as well as plating, doing physiological test and extracting chemicals from bacteria. |
Original/Discovery | Finding novel results, the results were previously unknown | Yes, because we received the opportunity to investigate a hypothesis that nobody knows the answer to yet. | Yes. We were doing an experiment that attempted to answer question that hadn’t been answered before. | Yes. We were acquainted with the scientific method, but the river ecosystem had unknown results. |
Relevance | References some sort of greater purpose, contributing to knowledge in some way | Yes. I felt that the work I was doing was actually determining real variables that affect the American River. | Yes. We dealt with real issues that affect the world today. Such as antibiotic resistance and common bacteria. | Yes. The American River is a big contributor in Sacramento. Understanding what is going on in the river and the ecosystem will benefit the community. We will be more responsible in how we use it. |
Iteration | References growth, building on the work of others, or repetition | Yes. because we were given the opportunity to do trials for our experiment | Yes. There was a trial and error for our lab. | Yes. Yes, not all of the experiments generated an expected result. Troubleshooting was always a major skill to look at what worked or didn’t work to proceed with the experiment. |
Collaboration | Working with peers, faculty, staff | Yes, because I got to do experiments with my peers, we collected the data and analyzed it. | Yes, a group of my peers and I conducted our own experiment. | Yes. We were expected to…learn how to work in a group and be able to switch around working in the lab. |
Independence | Working on experiment by themselves | Yes, because we are finding our own results. | Yes. This was the first class that I really had to conduct my own experiment from scratch. | Yes. I am …forming my own hypothesis. I am also interpreting my data, and these are not results that the professor has already set up. |
Personal Benefits | Gaining content knowledge or skills that will be beneficial in later classes or careers | Yes. Lab constantly challenges me to further my knowledge. It teaches me hands on skills that I would never have learned without lab. | Yes. I think what we did …helped us to build a stronger foundation for more in-depth biology classes to come. | Yes. …Also, I was able to learn new lab techniques that are crucial for a career. |
Resilience and Failure | References an experiment not working but understanding that is part of science | Yes…Although the results did not come out the way we wanted, we can still learn from it and our mistakes. | Yes. because none of my results were ever correct or neat on the first try. I experienced a lot of problems a scientist would come across in a lab setting. | Yes. This lab course was challenging and informative as it has taught me to be confident in myself even though I was not achieving the goals I wanted, but it taught me to adapt and solve problems and then results aligned with those goals. |
Limited | Experience was missing something the student felt should have been included | Not necessarily because our only job was to investigate the species' richness. | Maybe. The time and resource constraints were difficult to dedicate to a hypothesis in depth. | Yes. To some degree. I would have liked to be involved in the process of plating the bacteria and worms. |
DISCUSSION
The higher discovery/relevance and iteration LCAS scores from our advanced classes, relative to lower division courses, are consistent with what we would expect from a curriculum of scaffolded CUREs. Scores from our advanced classes were higher in the discovery/relevance and iteration LCAS constructs compared with scores from our lower division courses, and also higher than other courses reported in the literature (Table 9; Beck et al., 2023). This is especially notable given that the results were not from a single class, but rather from five different classes with five different instructors. Several factors may have contributed to these higher scores. Smaller class sizes and students’ prior knowledge and skills could have allowed faculty to genuinely embed a greater amount of authentic work in the course. Sathy et al., (2020) also observed higher scores in their advanced research methods for a psychology course (taught by the same instructor as their introductory research methods course) and suggested smaller class sizes as a possible cause. An additional factor might be consistent messaging from faculty teaching at the advanced level, all of whom participated in the SIRIUS FLC professional development activities. By contrast, lower medians and greater variability in scores for intermediate classes may have been due to the fact that fewer of the lab instructors for these courses participated in the SIRIUS FLC. A third possible factor is that students in the advanced classes may have previously participated in introductory or intermediate level SIRIUS CUREs, and repeated experiences with CUREs might have helped students recognize when they were engaging in relevant discovery and iteration.
Reference | Course type | Percent of range | ||
---|---|---|---|---|
Collaboration | Discovery | Iteration | ||
Corwin, Runyon et al., (2015) | Biology courses with CUREs (intro and upper division) | 88 | 81 | 80 |
Corwin, Runyon et al., (2015) | Biology courses without CUREs (intro and upper division) | 87 | 69 | 74 |
Allen et al., (2021) | Chemistry (upper division, Analytical Chemistry) | 92 | 81 | 81 |
Sathy et al., (2020) | Introductory Statistics | 83 | 79 | 76 |
Sathy et al., (2020) | Research Methods (Psychology) | 76 | 85 | 81 |
Sathy et al., (2020) | Advanced Research Methods (Psychology) | 88 | 91 | 85 |
Cruz et al., (2020) | Organic Chemistry (large enrollment, lower division) | 72 | 74 | 72 |
Kearney (2022) | Introductory Biology | 90a | 85a | 78a |
Goodwin et al., (2021) | Introductory Biology–Inquiry | 81 | 77 | 74 |
Goodwin et al., (2021) | Introductory Biology–CURE | 87 | 87 | 88 |
This study | Introductory Biology courses | 90 | 80 | 77 |
This study | Intermediate-level Biology courses | 87 | 77 | 72 |
This study | Advanced Biology courses | 100 | 93 | 92 |
The lower LCAS scores for iteration observed in intermediate classes compared with introductory and advanced courses underscore the value of LCAS scores as a tool for assessing individual course and program impacts. The lower iteration scores in intermediate classes were consistent with the CURE designs course instructors described to the project coordinators. Introductory and advanced CUREs were typically longer in duration and provided more opportunities for repetition and revision than intermediate CUREs. This agreement supports Beck and co-authors' assertion that the utility of the LCAS lies in helping us identify what students are doing in their laboratory classes (Beck et al., 2023).
Interestingly, however, iteration did not appear to contribute strongly to introductory- or intermediate-level students’ perceptions that they were doing real science, based on answers to the open-ended question. While an actual lack of iteration could explain these results for intermediate-level courses, introductory-level courses experienced iteration but did not mention it as an aspect of real science. Advanced-level courses also experienced iteration in their CUREs and students mentioned this code fairly frequently (in ∼30% of answers) as an aspect of real science. As noted earlier, all of the instructors in the advanced-level courses participated in professional development that included an introduction to the elements of a CURE, and they may have emphasized iteration as an important step not only in their CURE but also in “real science.” Based on Rowland and colleagues’ (2016) review of authentic science definitions, we would not expect instructors to emphasize iteration or collaboration as aspects of real science, as they were rarely included as a characteristic of authenticity. Collaboration had an even more striking mismatch between frequency of students mentioning it in the open-ended question and scores on the LCAS, even at the advanced level. This suggests that, especially for introductory- and intermediate-level students, discovery/relevance and scientific practices are the CURE elements (as defined in Auchincloss 2014) most likely to contribute to students’ perception that they are doing real science.
Student answers to our open-ended question about whether they did real science in their courses also suggest that students in advanced courses were not only experiencing more sophisticated CURE curricula, but also developing broader views about what it means to do authentic science. Consistent with other studies of student and faculty perceptions of what constitutes authentic science (Spell et al., 2014; Rowland et al., 2016; Wiggins et al., 2021), scientific practices were the most frequent reason provided by students at any level. When disaggregating scientific practice in our study, however, students from advanced classes more frequently referenced practices that involved scientific thinking (as opposed to physical lab or field techniques) than students from intermediate and introductory courses. This observation is consistent with curriculum analysis of SIRIUS courses at the three levels, which indicated that students engaged in more peripheral tasks at the introductory- and intermediate-level and more central tasks in advanced-level courses (Fajardo, 2022). While students at all levels were participating in real research, the degree of participation was intentionally scaffolded, so students progressed into deeper cognitive involvement in the research activities as they progressed through the curriculum.
Disaggregating relevance and discovery in open-ended responses revealed that students’ perceptions of relevance were mostly consistent across levels, but students from the advanced classes mentioned discovery nearly four times as often as students in introductory or intermediate classes. Research in each course had an element of novelty, but the degree may have differed. For example, BIO 1 students manipulated fertilizer levels in microcosms filled with water and sediment from the river by campus. While the instructor did not know what to expect with the faunal assemblages in these specific samples, students may have suspected that this was something that had been done before in other rivers. Also, instructor communication may have played a role, with advanced-level instructors placing greater emphasis on the novelty of their work than introductory or intermediate instructors. Interestingly, for our advanced students, the idea that they were doing something “novel” or investigating a question that no one had answered appears to have supported their work’s authenticity more than the potential for their work to be meaningful to a broader community. This result suggests that considering relevance and discovery as distinct items (as opposed to combining them, as the LCAS does) may be useful when modeling and testing positive effects of CURE elements.
Our study found that students commented more about failure in the advanced courses than the introductory or intermediate courses. Failure and frustration, with the opportunity to troubleshoot, have emerged as useful characteristics of CUREs (Lopatto et al., 2020), as well as reasons students perceive their CURE curricula to represent authentic research (Rowland et al., 2016; Goodwin et al., 2021; Wiggins et al., 2021). Both Goodwin et al., (2021) and our study saw examples of students saying the research was “real” because it didn’t work. Unexpected challenges lend a sense of authenticity and create a contrast with “cookbook” labs that have been designed to reliably illustrate certain outcomes. Another aspect of failure that appeared in our study and others is students’ realization that failure is a part of real research—something they will encounter in the future (Rowland et al., 2016; Goodwin et al., 2021). Our results support those of a number of studies that have found that students value the opportunity to fail, troubleshoot, and repeat an experiment (Rowland et al., 2016; Lopatto et al., 2020; Wiggins et al., 2021). Students recognize that this cycle helps them learn, is valuable preparation for future research positions, and can be enjoyable when it leads to success. Conversely, a small number of students felt like it was not real science if they did not have time to repeat their work until they were successful in both our study and Goodwin and colleagues’ (2021). Overall, however, the ability to fail and repeat appears to be a positive benefit of CUREs, especially in advanced courses.
Students’ overall perception that they were doing real science in the SIRIUS Project CUREs, regardless of their rationales, is encouraging and important for several reasons. Research suggests that student perceptions of specific CURE components correlate with desired student outcomes. For instance, studies have shown that perceptions of relevance and discovery (as measured by the LCAS) are positively correlated with a sense of project ownership (Corwin et al., 2018, Cooper et al., 2019). Cooper et al., (2019) used the LCAS to evaluate two sections of a course that differed only in the degree of relevance and novel discovery and found that students perceived this difference and also reported greater project ownership in the section with relevance and discovery in the design. A sense of project ownership has been associated with outcomes such as persistence in science (Hanauer et al., 2016), suggesting the inclusion of these CURE components could have both short and long-term influences. Finally, iteration and failure can help students develop stronger experimental design skills even in laboratory courses that lack strong relevance and discovery components (Lansverk et al., 2020).
In addition to the psychosocial and cognitive benefits described above, students who recognize the authenticity or importance of their work may be more likely to highlight it when pursuing future academic or career opportunities. Some faculty in advanced SIRIUS courses explicitly addressed how students might include their CURE research in resumes, graduate school applications, or interviews, thus providing a more diverse student population with access to advanced academic and career paths. Another potential benefit that could be explored is that understanding characteristics of real research (like failure and iteration) might ease the transition into graduate work for students, through changing expectations and building resilience.
Limitations
The greatest limitations of this study involved data collection and sample sizes, which also influenced the application of a cross-sectional, rather than longitudinal, design. SIRIUS courses were implemented over a three-year period, with introductory courses introduced first and advanced courses introduced last, providing more time to collect data in the early-implementation courses. All advanced courses, with the exception of one, ran only once during the evaluation time frame. This was in part due to wildfires, which closed the campus during one semester that surveys were being administered to advanced students. Advanced course section sizes were small to begin with, and at the time, these courses were run only once per year, which further limited data collection. However, the advanced-level dataset included student responses from four to five distinct classes taught by different instructors, which may have served as a strength to the study design (i.e., more repeated treatments). Limited numbers, however, prevented us from addressing other factors that could have influenced student’s perceptions, such as the number and combination of SIRIUS CURE courses completed. Our data do suggest that each level is composed of students that were similar demographically, and that these CUREs are impacting students with different identities in a similar manner; however, limited numbers across all sections prevented a thorough exploration of demographic variables that could have influenced perceptions.
Another limitation is the lack of uniformity in training for the faculty involved in teaching the SIRIUS courses (Table 2). Differences in instructors’ degree of training and level of participation in CURE designs could affect students’ perceptions of CUREs. Thus, observed differences in student perceptions across course levels may partially stem from instructor training and “buy-in.” Specifically, the high LCAS scores and sophisticated responses to the “real science” question in advanced courses could be explained partly by the courses being taught solely by SIRIUS FLC participants, all of whom were tenure-track faculty. For intermediate and introductory courses, some lecturers and GTAs participated in SIRIUS professional development and course-design activities. However, introductory courses had a greater percentage of lecturers and GTAs who did not participate in the faculty learning community, and thus may have presented and facilitated the CUREs differently. That said, all course coordinators for multi-section courses were part of the FLC and performed some training for the lecturers and GTAs teaching the CUREs.
Finally, we reiterate that differences observed between levels cannot be attributed to a single factor. Characteristics of the CUREs, such as length of time (Table 2) and degree of autonomy differed between levels. Students’ accumulated knowledge and skills (including experience gained in other SIRIUS courses) differed between levels, as well. Last, class sizes and the degree of connection between lecture and lab sections differed between courses. While we are unable to pinpoint exact causes for differences, we are nonetheless encouraged by students’ perception of authenticity, especially in the advanced levels.
Future Directions
This study was largely motivated by our aim to assess the SIRIUS curricula designed by faculty in our multi-year learning community to help inform a second phase of the project. In the newly-funded SIRIUS II project, we are expanding our model to 10 STEM disciplines and five institutions – one four-year institution and four community colleges - united around a shared vision to train students with authentic and interdisciplinary experiences. In the assessment of SIRIUS II, we have the opportunity to explore questions that arose from this work, such as the relationship between the perceived authenticity and student outcomes or benefits. As the SIRIUS project was initiated to solve a problem related to limited research opportunities and inequitable access to authentic experiences, we also seek to determine whether the SIRIUS program is shifting the demographics of students who perform extracurricular research to include more students from historically and currently marginalized populations.
CONCLUSION
Our study indicates that students progressing through a multi-level CURE program recognize the authenticity of their laboratory work and perceive engaging in activities related to Collaboration, Discovery/Relevance, and Iteration. They also perceive an increased degree of independence and more opportunities to fail as they progress from introductory to advanced coursework. With this report, we add to the literature on CUREs, and highlight the potential benefits of a scaffolded CURE curriculum. We also confirm previous findings that integrating autonomy and opportunities for failure are especially salient elements that influence students’ perceptions about science/research authenticity. Lastly, we highlight the benefits of using a triangulation mixed methods design, with different instruments addressing similar questions, to support findings and understand nuanced data. While our methods provided us with useful data about student perceptions of their CUREs, future work is needed to understand the influence of positive (or negative) student perceptions of authenticity on other outcomes. The question remains, do students who perceive their lab experiences as authentic science benefit more from CUREs than students who do not?
ACKNOWLEDGMENTS
The authors thank Dr. Beth Schussler and Dr. Maryrose Weatherton for their thoughtful comments and feedback on versions of this paper. We thank the editor and the reviewers for their helpful feedback throughout the revision process. We also thank the National Science Foundation and the William M. Keck foundation for funding the SIRIUS Project.